Iron (and how regulating your levels may be an important anti-ageing tool)
- Healthfully Myself
- May 30, 2024
- 15 min read
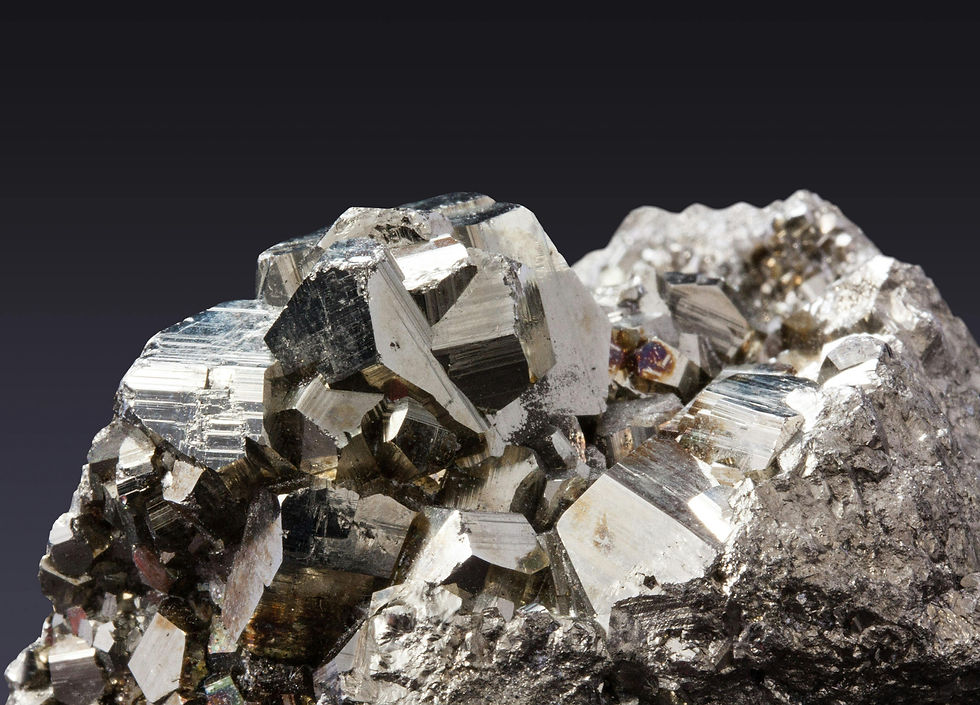
Iron is one of the most abundant metals in the human body and is distributed in almost all
tissues and organs (1). Its function as a transition metal is critical for various physiological functions, including oxygen transport, DNA synthesis, and energy production. Maintaining iron homeostasis is critical, and iron deficiency and overload can have negative consequences. However, iron is undisputedly indispensable, it accumulates with age, and is associated with many age-related diseases; it is shown to shorten the lifespans of several organisms, even within the medically deemed acceptable ranges (2). This article aims to explore the mechanisms through which iron impacts health, and the associated risks, with a particular focus on iron’s role in the ageing process, and lifespan.
The importance of iron
Iron is so essential for growth and maintenance that all organisms, from bacteria and algae to mammals, have evolved ways to collect and store iron from the environment. The biological importance of iron is largely attributable to its chemical properties as a transitional metal (4). In humans, iron is an essential component of many key enzymes and plays an important role in several important physiological processes due to its reactivity; its ability to accept or donate electrons. Key processes include oxygen transport, DNA synthesis and repair, and the maintenance of mitochondrial function (3). However, its reactivity also gives it the ability to be damaging within the body when it reacts with hydrogen peroxide, leading to the formation of the highly reactive and toxic free radical, hydroxyl (this process is known as the Fenton reaction). (2). Most organisms, including humans, have no built-in means of eliminating excess iron, which we will see later on can be problematic.
The roles of iron
Oxygen transport - Iron is a key component of haemoglobin and myoglobin, which are necessary for transporting oxygen from the lungs to tissues and organs (5).
Immune function - Iron is crucial for the development and function of immune cells, aiding in the body’s ability to fight infections (5).
Energy production - Iron is a component of cytochromes in mitochondria, essential for cellular respiration and energy (ATP) production (5).
Cognitive health - Adequate iron levels are essential for brain function, and are associated with improved cognitive performance and brain development (in children) (5).
DNA synthesis and repair - Iron is a cofactor for enzymes involved in DNA synthesis and repair, which is crucial for cell replication and function (5).
Skeletal muscle function - Iron homeostasis significantly contributes to the effective functioning of skeletal muscles and may help preserve muscle strength in older adults (6).
Iron deficiency
Iron deficiency affects 20% to 50% of the world's population, making it the most common nutritional deficiency (7). Iron deficiency is defined as a condition in which there are no mobilisable iron stores which results in a reduced supply of iron to tissues. In mild cases of iron deficiency, some functional changes may be noticeable, but the deficits appear to occur with the development of anaemia (8). A variety of impairments can be noted in iron deficiency including:
Impaired cognitive development (9)
Suboptimum immunity (10)
Suboptimum work capacity (including severe fatigue and weakness (11)
A variety of adverse outcomes during pregnancy (for mother and baby) (12)
Developmental delays in children (13)
Iron overload
While iron deficiency is a significant concern, iron overload can also pose health risks, and more serious events occur due to the toxicity of iron overload (14). Iron overload can be a consequence of various iron metabolism disorders, chronic liver disease, or simply a daily high dietary iron intake (14). Any of the above reasons for excessive iron absorption can lead to iron accumulation in organs, potentially causing a multitude of serious problems such as (15):
Decreased immune function
Heart problems
Type 1 diabetes and metabolic syndrome
Bone loss
Kidney disease
Liver disease
Neurotoxicity
Iron and ageing
The above sections have focused on iron levels outside of the acceptable range that is often indicated in your blood test results. However, now we will turn to iron’s role in the ageing process which in many cases has been observed in individuals within the acceptable range.
Iron and oxidative stress
Most iron in cells is bound to proteins and other molecules. In humans, these are haemoglobin (a protein in our red blood cells), ferritin (stores iron), and transferrin (allows iron to travel in the blood) (2). In theory, these molecules safely store iron and prevent it from interacting with other molecules in the body; protecting the body from its high reactivity. However, ferritin can be damaged causing it to release free iron, causing the Fenton reaction that we have discussed, forming free radicals which leads to oxidative stress. This oxidative stress can lead to organ damage.
The oxidative damage theory is considered a major factor behind the aging process and is believed to contribute to the development of age-related diseases (16,17). The oxidative damage theory believes that reactive oxygen species (ROS) damage molecules in the body at the level of the mitochondria. Over time this causes extensive mitochondrial and cellular dysfunction, which leads to aging (18). As we now know, excessive iron can increase ROS production which leads to oxidative damage to cells (1). The problem is compounded as when we age, iron content increases, resulting in increased ROS production (1)
Oxidative damage to cells eventually leads to cellular senescence. Senescent cells accumulate during aging and have been implicated in promoting a variety of age-related diseases (19).
Of course you may ask, what comes first the chicken or the egg? Does excess iron accumulated because of ROS or does excess iron cause ROS? ……Well, this is yet to be determined conclusively, but there are several other reasons to think that iron is a driver of aging. These will be covered in subsequent sections!
Iron Metabolism and Life Expectancy
A recent report suggests that high levels of iron in the blood are associated with a reduced healthy lifespan (20). The observed increased iron content with age, and increased ROS production, has not only been indicated in disease manifestations but an overall reduction in lifespan (1). This idea is supported by observational evidence that iron restriction slows the aging process (21,22). In 2020, one such study found that higher genetically predicted serum iron was associated with shorter lifespans (20). This finding was confirmed and extended in a different group of people, showing that both high serum iron and transferrin saturation are associated with increased all-cause mortality (23). Finally, a huge meta-analysis added high ferritin levels as a risk factor for all-cause mortality (24). As a consequence, ferritin, transferrin saturation, and iron levels (markers of iron metabolism) have now been associated with predicted mortality or longevity. In fact, one theory behind why women tend to live longer than men is that they typically have lower iron levels until they reach menopause.
Coming at it from another angle, many compounds and interventions indicated to increase lifespan (geroprotectors) can be explained by the effects they have on iron absorption, excretion, and metabolism; usually by blocking dietary iron absorption, or binding iron and removing it (2). For example:
In insect and animal models, it has been found that curcumin reduces iron levels by binding (chelates) iron so it can be excreted (25).
In humans, long-term aspirin use is believed to contribute to chronic iron loss through its common side effect of gastric bleeding (26). Indeed, observational studies have shown that regular aspirin users have lower serum ferritin. Aspirin has also been found to reduce the risk of cancer (27).
Another well-established geoprotector is calorie restriction, which has consistently been shown to slow the intrinsic rate of aging and increase both the median and maximum life span in a variety of species (28,29). Calorie restriction has been found to protect against age-related iron accumulation, which we know is associated with a shorter lifespan and disease (30).
Collectively, the evidence indicates a strong positive correlation between iron accumulation and aging. Control of body iron stores so that they remain at the lower end of the normal range may be an important, lifespan- and healthspan-extending intervention.
Neurodegenerative diseases
Neurodegenerative diseases are caused by the loss of neurons and will worsen over time. There is enough evidence to prove that iron is indeed a key factor in neurodegenerative diseases (31). In recent years, there has been increasing evidence that the imbalance of iron homeostasis in the brain may be at the core of many age-related neurodegenerative diseases. It is indicated that progressive iron accumulation in the brain produces free radicals (via the Fenton reaction), which is thought to be the core of age-related diseases such as Alzheimer’s, Parkinson’s disease, and other neurodegenerative disorders (32,33).
Cardiovascular health
It is well-established that oxidative stress has an integral role in the pathogenesis of atherosclerosis. The ease with which iron is easily oxidised and reduced makes it able to generate oxidised low-density lipoproteins (LDL) and oxygen-free radicals that can contribute to vessel wall damage and atherosclerosis. Collective data from experimental studies, including animal studies reveal that iron chelators (agents which remove iron) prevent the oxidation of LDL and vessel wall damage (34,35).
Epidemiological studies examining the relationship between iron status and coronary hearth disease (CHD) have produced conflicting results. Although the evidence is still inconclusive, several mechanisms explaining how iron can contribute to cardiovascular disease (CVD) have been proposed. Most of which are a result of the Fenton reaction. Iron’s role in certain enzyme systems of the body has also been proposed to contribute to endothelial dysfunction; and subsequent CVD risk (36).
Substantial observational evidence supports a link between higher iron levels and CVD. One of the first studies in 1992 examining this relationship, found that men with ferritin levels within the high-normal range had an increased risk of myocardial infarction (aka. "heart attack") compared with men with lower serum ferritin (37). This association has also been observed in conditions that cause chronic iron overload. Those with the HFE hereditary haemochromatosis mutation have an increased occurrence of CHD (38,39). Conversely, several studies have found that blood donors (who have less stored iron) have a reduced risk of heart attack (40,41). In clinical studies, iron deposits have been detected in coronary plaques in patients with atherosclerotic lesions (42). The plaques of patients in more advanced stages of the disease showed higher iron concentrations and risk of cap rupture compared with those in less advanced stages (43). Additionally, iron chelation therapy has been found to be beneficial to these patients (44). In all of these studies, the participants with some form of CVD had higher serum ferritin levels, but mostly within the accepted range.
However, there still exist contradictions to these findings. A systematic review and meta-analysis of 17 studies found no significant association between the other markers of iron status and CHD. (45). In fact it even found that high transferrin level saturations were associated with a lower risk of coronary artery disease or myocardial infarction.
Important note:
This evidence is specific to individuals with very specific types of CVD. For certain people, in particular those with heart failure with reduced ejection fraction, studies have shown that iron deficiency is common, and correcting this deficiency improves outcomes (46). Therefore, it is extremely important to consult a medical professional if you are suffering from CVD and are concerned about your iron levels.
Cancer risk
Epidemiological studies have demonstrated an association between excess iron and increased incidence and risk of certain cancers, while experimental studies have implicated iron in cancer initiation, tumour growth, and metastasis. (47). It is proposed that iron’s role in promoting the formation of ROS causes carcinogenesis, through the damage it does to cells, and in the case of cancer; to DNA (48). This effect has been has been shown in animal and human models. Iron, in particular haem-iron (49), is suggested as a risk factor for many types of cancer (50), including liver (51), colorectal (52), breast (53), and lung cancer (54).
One of the initial studies associating body iron levels and cancer, was conducted in 1994 in a group of 14,000 participants (55). A key finding was that participants with higher transferrin saturation levels were at higher risk of cancer than participants with lower saturation levels. This finding has been supported by later studies (56). The risk was increased even among participants in the higher but still considered normal transferrin saturation range (15 – 50%).
Studies examining cancer in individuals with iron overload conditions further support the association between iron and increased cancer risk. It has been observed that individuals with hereditary hemochromatosis are at an increased risk of developing several cancers, including liver, colon, rectal, prostate, and breast cancers (47). The liver plays a critical role in iron metabolism therefore, conditions where iron is in excess are particularly detrimental to the liver. The risk of liver cancer is particularly high in these individuals (20 to 200 times higher than those without any of these conditions) (57,58). The majority of uncontrolled hemochromatosis patients suffer from cirrhosis and hepatocarcinoma (47).
Conversely, blood donation, which reduces body iron, is associated with a lower cancer risk (59,60). A study in patients with advanced arterial diseases demonstrated that iron reduction by phlebotomy (aka. “bloodletting”) lowered cancer risk and mortality (60). It was found that this group of men (who had iron within the normal range) had a 37% lower risk of developing cancer, and those who did develop cancer had a survival rate around twice that of the control group.
Both iron overload and iron depletion can be utilised in anticancer therapies. Therapies usually either involve depriving cancer cells of iron, or the reverse. Tumour cells have a higher metabolic and proliferation rate than normal cells, therefore their demand for iron is higher (61). Limiting iron availability is used to change the metabolism of tumour cells; to the detriment of the cells. Conversely, excess iron is used to selectively deliver toxic levels of ROS to cancer cells (47). In this strategy, tumour cells are supplied with excess iron, causing high levels of unstable iron, ultimately generating large amounts of ROS to eliminate tumour cells (61).
Practical strategies to control iron
Given the potential risks associated with both iron deficiency and overload, managing iron levels is crucial. It is strongly suggested that you and your doctor determine the optimum iron level for your health. Here are some strategies that can be used to control your iron levels:
Regular monitoring - Regular blood tests to monitor iron levels, including serum ferritin and transferrin saturation, can help detect imbalances early.
Dietary management - Consuming a balanced diet that includes both animal-based (haem) and plant-based (non-haem) iron sources can help maintain appropriate iron levels. Vitamin C enhances the absorption of non-haem iron. It is recommended that iron supplements are only used under medical supervision, to avoid the risk of overload (it also pays to check your multivitamins for added iron!).
Iron chelation compounds, medications, or supplements - These remove extra iron from your body, and can be over-the-counter supplements, or prescription medications. Iron chelators can also be injected by a healthcare provider in extreme cases.
Therapeutic phlebotomy or regular blood donation - These procedures reduce iron levels by removing blood (including iron) from your body.
Final remarks
Iron is undeniably a vital element for human health however, the relationship between iron levels and health is complex and nuanced. While iron deficiency remains a significant global health issue, iron overload poses arguably more serious risks. Excessive iron can lead to oxidative stress, contributing to organ damage, accelerated aging, and various age-related diseases. Studies suggest that even within medically acceptable ranges, higher iron levels may be associated with shorter lifespans and increased disease risks, highlighting the need for a balanced approach to iron management. While there is no “perfect” iron level, after reading the research, I will be aiming to maintain my iron levels within the lower end of the normal range.
*Disclaimer*
The information presented in this article is for educational purposes only and based on my interpretation of the research. Individuals with specific health concerns or dietary restrictions should consult with qualified healthcare professionals or registered dietitians for personalised guidance and recommendations tailored to their individual needs and circumstances.
References
1. Tian Y, Tian Y, Yuan Z, Zeng Y, Wang S, Fan X, Yang D, Yang M. Iron metabolism in aging and age-related diseases. Int. J. Mol. Sci. 2022; 23(7):3612. https://doi.org/10.3390/ijms23073612
2. Mangan D. Iron: an underrated factor in aging. Aging (Albany NY). 2021;13(19):23407-23415. https://doi.org/10.18632/aging.203612
3. Hentze MW, Muckenthaler MU, Andrews NC. Balancing acts: Molecular control of mammalian iron metabolism. Cell. 2004;117(3):285-297. https://doi.org/10.1016/s0092-8674(04)00343-5
4. Okazaki Y, Hino K. Iron and cancer: A Special Issue. Cancers. 2023; 15(7):2097. https://doi.org/10.3390/cancers15072097
5. Beard JL. Iron biology in immune function, muscle metabolism and neuronal functioning. J Nutr. 2001;131(2S-2):568S-580S. https://doi.org/10.1093/jn/131.2.568S
6. Stugiewicz M, Tkaczyszyn M, Kasztura M, Banasiak W, Ponikowski P, Jankowska EA. The influence of iron deficiency on the functioning of skeletal muscles: experimental evidence and clinical implications. Eur J Heart Fail. 2016;18(7):762-773. https://doi.org/10.1002/ejhf.467
7. Stoltzfus RJ. Iron-deficiency anemia: Reexamining the nature and magnitude of the public health problem. Summary: implications for research and programs. J Nutr. 2001;131(2S-2):697S-701S. https://doi.org/10.1093/jn/131.2.697S
8. Wood RJ, Ronnenberg A. Iron. In: Shils ME, Shike M, Ross AC, Caballero B, Cousins RJ, editors. Modern Nutrition in Health And Disease. 10th ed. Baltimore: Lippincott Williams & Wilkins; 2005. pp. 248–70.
9. Beard JL, Connor JR. Iron status and neural functioning. Annu Rev Nutr. 2003;23:41-58. https://doi.org/10.1146/annurev.nutr.23.020102.075739
10. Failla ML. Trace elements and host defense: recent advances and continuing challenges. J Nutr. 2003;133(5 Suppl 1):1443S-7S. https://doi.org/10.1093/jn/133.5.1443S
11. Viteri FE, Torun B. Anemia and physical work capacity. In: Garby L, editor. Clinics in Hematology. Vol. 3. London: WB Saunders; 1974. pp. 609–26.
12. CDC. Breastfeeding Report Card, United states: Outcome indicators (Publication, from Centers for Disease Control and Prevention, National Immunization Survey. 2010. http://www.cdc.gov/breastfeeding/data/index.htm
13. Saloojee H, Pettifor JM. Iron deficiency and impaired child development. BMJ. 2001;323(7326):1377-1378. https://doi.org/10.1136/bmj.323.7326.1377
14. Santra S, Agrawal D, Kumar S, Mishra SS. Incidence and prevalence of chronic iron poisoning and it’s management: A review. Int. J. Pharma Bio Sci. 2014;5:722–737
15. Sousa L, Oliveira MM, Pessôa MTC, Barbosa LA. Iron overload: Effects on cellular biochemistry. Clin Chim Acta. 2020;504:180-189. https://doi.org/10.1016/j.cca.2019.11.029
16. McLean E, Cogswell M, Egli I, Wojdyla D, de Benoist B. Worldwide prevalence of anaemia, WHO Vitamin and Mineral Nutrition Information System, 1993-2005. Public Health Nutr. 2009;12(4):444-454. https://doi.org/10.1017/S1368980008002401
17. López-Otín C, Blasco MA, Partridge L, Serrano M, Kroemer G. The hallmarks of aging. Cell. 2013;153(6):1194-1217. https://doi.org/10.1016/j.cell.2013.05.039
18. Buffenstein R, Edrey YH, Yang T, Mele J. The oxidative stress theory of aging: embattled or invincible? Insights from non-traditional model organisms. Age (Dordr). 2008;30(2-3):99-109. https://doi.org/1007/s11357-008-9058-z
19. Regulski MJ. Cellular senescence: What, why, and how. Wounds. 2017;29(6):168-174.
20. Timmers PRHJ, Wilson JF, Joshi PK, Deelen J. Multivariate genomic scan implicates novel loci and haem metabolism in human ageing. Nat Commun. 2020;11(1):3570. Published 2020 Jul 16. doi:10.1038/s41467-020-17312-3
21. Xu J, Marzetti E, Seo AY, Kim JS, Prolla TA, Leeuwenburgh C. The emerging role of iron dyshomeostasis in the mitochondrial decay of aging. Mech Ageing Dev. 2010;131(7-8):487-493. https://doi.org/10.1016/j.mad.2010.04.007
22. Polla AS, Polla LL, Polla BS. Iron as the malignant spirit in successful ageing. Ageing Res Rev. 2003;2(1):25-37. https://doi.org/10.1016/s1568-1637(02)00048-x
23. Moksnes MR, Graham SE, Wu KH, et al. Genome-wide meta-analysis of iron status biomarkers and the effect of iron on all-cause mortality in HUNT. Commun Biol. 2022;5(1):591. https://doi.org/10.1038/s42003-022-03529-z
24. Daghlas I, Gill D. Genetically predicted iron status and life expectancy. Clin Nutr. 2021;40(4):2456-2459. https://doi.org/10.1016/j.clnu.2020.06.025
25. Jiao Y, Wilkinson J 4th, Christine Pietsch E, et al. Iron chelation in the biological activity of curcumin. Free Radic Biol Med. 2006;40(7):1152-1160. https://doi.org/10.1016/j.freeradbiomed.2005.11.003
26. Mascitelli L, Pezzetta F, Sullivan JL. Aspirin-associated iron loss as an anticancer mechanism. Med Hypotheses. 2010;74(1):78-80. https://doi.org/10.1016/j.mehy.2009.07.047
27. Rothwell PM, Fowkes FG, Belch JF, Ogawa H, Warlow CP, Meade TW. Effect of daily aspirin on long-term risk of death due to cancer: analysis of individual patient data from randomised trials. Lancet. 2011;377(9759):31-41. https://doi.org/10.1016/S0140-6736(10)62110-1
28. Spindler SR. Caloric restriction: from soup to nuts. Ageing Res Rev. 2010;9(3):324-353. https://doi.org/10.1016/j.arr.2009.10.003
29. Colman RJ, Anderson RM, Johnson SC, et al. Caloric restriction delays disease onset and mortality in rhesus monkeys. Science. 2009;325(5937):201-204. https://doi.org/10.1126/science.1173635
30. Wei S, Shi W, Li M, Gao Q. Calorie restriction down-regulates expression of the iron regulatory hormone hepcidin in normal and D-galactose-induced aging mouse brain. Rejuvenation Res. 2014;17(1):19-26. https://doi.org/10.1089/rej.2013.1450
31. Rouault TA. Iron on the brain. Nat Genet. 2001;28(4):299-300. https://doi.org/10.1038/91036
32. Zecca L, Youdim MB, Riederer P, Connor JR, Crichton RR. Iron, brain ageing and neurodegenerative disorders. Nat Rev Neurosci. 2004;5(11):863-873. https://doi.org/10.1038/nrn1537
33. Gerlach M, Ben-Shachar D, Riederer P, Youdim MB. Altered brain metabolism of iron as a cause of neurodegenerative diseases?. J Neurochem. 1994;63(3):793-807. https://doi.org/10.1046/j.1471-4159.1994.63030793.x
34. Sullivan JL. Iron and the sex difference in heart disease risk. Lancet. 1981;1(8233):1293-1294. https://doi.org/10.1016/s0140-6736(81)92463-6
35. Howes PS, Zacharski LR, Sullivan J, Chow B. Role of stored iron in atherosclerosis. J Vasc Nurs. 2000;18(4):109-116. https://doi.org/10.1067/mvn.2000.111614
36. Cooper CE. Nitric oxide and iron proteins. Biochim Biophys Acta.1999;1411:290–309. https://doi.org/10.1016/S0005-2728(99)00021-3
37. Salonen JT, Nyyssönen K, Korpela H, Tuomilehto J, Seppänen R, Salonen R. High stored iron levels are associated with excess risk of myocardial infarction in eastern Finnish men. Circulation. 1992;86(3):803-811. https://doi.org/10.1161/01.cir.86.3.803
38. Lian J, Xu L, Huang Y, et al. Meta-analyses of HFE variants in coronary heart disease. Gene. 2013;527(1):167-173. https://doi.org/10.1016/j.gene.2013.06.034
39. Pardo Silva MC, Njajou OT, Alizadeh BZ, et al. HFE gene mutations increase the risk of coronary heart disease in women. Eur J Epidemiol. 2010;25(9):643-649. https://doi.org/10.1007/s10654-010-9489-6
40. Meyers DG, Strickland D, Maloley PA, Seburg JK, Wilson JE, McManus BF. Possible association of a reduction in cardiovascular events with blood donation. Heart. 1997;78(2):188-193. https://doi.org/10.1136/hrt.78.2.188
41. Salonen JT, Tuomainen TP, Salonen R, Lakka TA, Nyyssönen K. Donation of blood is associated with reduced risk of myocardial infarction. The Kuopio Ischaemic Heart Disease Risk Factor Study. Am J Epidemiol. 1998;148(5):445-451. https://doi.org/10.1093/oxfordjournals.aje.a009669
42. Stadler N, Lindner RA, Davies MJ. Direct detection and quantification of transition metal ions in human atherosclerotic plaques: evidence for the presence of elevated levels of iron and copper. Arterioscler Thromb Vasc Biol. 2004;24:949–54. https://doi.org/10.1161/01.ATV.0000124892.90999.cb
43. Gustafsson H, Hallbeck M, Norell M, Lindgren M, Engström M, Rosén A, et al.. Fe(III) distribution varies substantially within and between atherosclerotic plaques. Magn Reson Med. 2014;71:885–92. https://doi.org/10.1002/mrm.24687
44. Duffy SJ, Biegelsen ES, Holbrook M, et al. Iron chelation improves endothelial function in patients with coronary artery disease. Circulation. 2001;103(23):2799-2804. https://doi.org/10.1161/01.cir.103.23.2799
45. Das De S, Krishna S, Jethwa A. Iron status and its association with coronary heart disease: systematic review and meta-analysis of prospective studies. Atherosclerosis. 2015;238(2):296-303. https://doi.org/10.1016/j.atherosclerosis.2014.12.018
46. Savarese G, von Haehling S, Butler J, Cleland JGF, Ponikowski P, Anker SD. Iron deficiency and cardiovascular disease [published correction appears in Eur Heart J. 2023 May 7;44(18):1607]. Eur Heart J. 2023;44(1):14-27. https://doi.org/10.1093/eurheartj/ehac569
47. Torti SV, Manz DH, Paul BT, Blanchette-Farra N, Torti FM. Iron and cancer. Annu Rev Nutr. 2018;38:97-125. https://doi.org/10.1146/annurev-nutr-082117-051732
48. Toyokuni S, Mori T, Dizdaroglu M. DNA base modifications in renal chromatin of Wistar rats treated with a renal carcinogen, ferric nitrilotriacetate. Int J Cancer. 1994;57(1):123-128. https://doi.org/10.1002/ijc.2910570122
49. Cross A.J., Pollock J.R., Bingham S. Haem, not protein or inorganic iron, is responsible for endogenous intestinal N-nitrosation arising from red meat. Cancer Res. 2003;63:2358–2360.
50. Fonseca-Nunes A., Jakszyn P., Agudo A. Iron and cancer risk--a systematic review and meta-analysis of the epidemiological evidence. Cancer Epidemiol. Biomark. Prev. 2014;23:12–31. https://doi.org/10.1158/1055-9965.EPI-13-0733
51. Yu Y.C., Luu H.N., Wang R., Thomas C.E., Glynn N.W., Youk A.O., Behari J., Yuan J.M. Serum biomarkers of iron status and risk of hepatocellular carcinoma development in patients with nonalcoholic fatty liver disease. Cancer Epidemiol. Biomark. Prev. 2022;31:230–235. https://doi.org/10.1158/1055-9965.EPI-21-0754.
52. Chua A.C., Klopcic B., Lawrance I.C., Olynyk J.K., Trinder D. Iron: An emerging factor in colorectal carcinogenesis. World J. Gastroenterol. 2010;16:663–672. https://doi.org/10.3748/wjg.v16.i6.663
53. Huang X. Does iron have a role in breast cancer? Lancet Oncol. 2008;9:803–807. https://doi.org/10.1016/S1470-2045(08)70200-6
54. Tasevska N., Sinha R., Kipnis V., Subar A.F., Leitzmann M.F., Hollenbeck A.R., E Caporaso N., Schatzkin A., Cross A.J. A prospective study of meat, cooking methods, meat mutagens, heme iron, and lung cancer risks. Am. J. Clin. Nutr. 2009;89:1884–1894. https://doi.org/10.3945/ajcn.2008.27272
55. Stevens RG, Graubard BI, Micozzi MS, Neriishi K, Blumberg BS. Moderate elevation of body iron level and increased risk of cancer occurrence and death. Int J Cancer. 1994;56(3):364-369. https://doi.org/10.1002/ijc.2910560312
56. Wu T, Sempos CT, Freudenheim JL, Muti P, Smit E. Serum iron, copper and zinc concentrations and risk of cancer mortality in US adults. Ann Epidemiol. 2004;14(3):195-201. https://doi.org/10.1016/S1047-2797(03)00119-4
57. Elmberg M, Hultcrantz R, Ekbom A, et al. Cancer risk in patients with hereditary hemochromatosis and in their first-degree relatives. Gastroenterology. 2003;125(6):1733-1741. https://doi.org/10.1053/j.gastro.2003.09.035
58. Fargion S, Valenti L, Fracanzani AL. Hemochromatosis gene (HFE) mutations and cancer risk: expanding the clinical manifestations of hereditary iron overload. Hepatology. 2010;51(4):1119-1121. https://doi.org/10.1002/hep.23541
59. Merk K, Mattsson B, Mattsson A, Holm G, Gullbring B, Björkholm M. The incidence of cancer among blood donors. Int J Epidemiol. 1990;19(3):505-509. https://doi.org/10.1093/ije/19.3.505
60. Zacharski LR, Chow BK, Howes PS, et al. Decreased cancer risk after iron reduction in patients with peripheral arterial disease: results from a randomized trial. J Natl Cancer Inst. 2008;100(14):996-1002. https://doi.org/10.1093/jnci/djn209
61. Guo Q, Li L, Hou S, et al. The Role of Iron in Cancer Progression. Front Oncol. 2021;11:778492. https://doi.org/10.3389/fonc.2021.778492
Comments